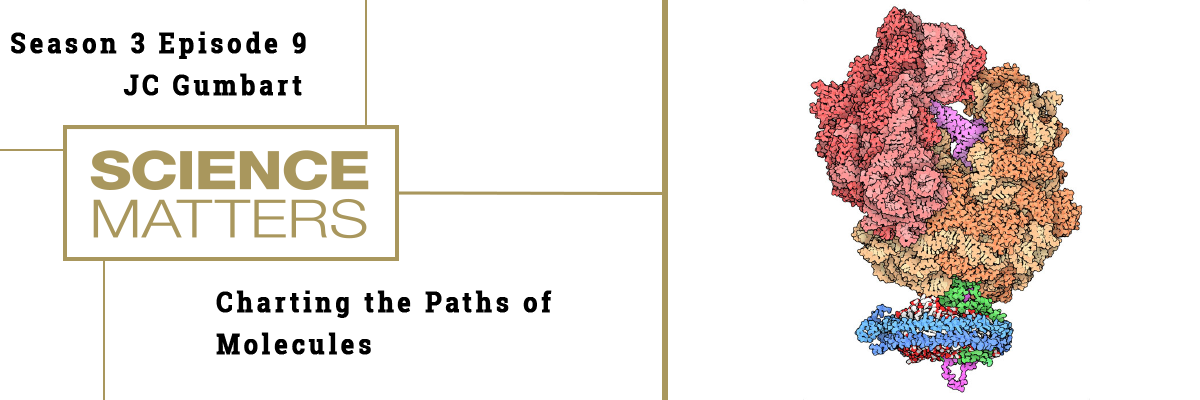
Download this episode
Cosmologists use computers simulations to recreate the birth of stars and black holes. James “JC” Gumbart, an associate professor in the School of Physics, goes in the other direction: He uses powerful hardware and software to chart the possible paths of molecules like proteins in hopes of finding solutions to problems like antibiotic-resistant bacteria.
(Upbeat music)
Renay San Miguel: Hello and welcome to ScienceMatters, the podcast of the Georgia Tech College of Sciences. I’m Renay San Miguel.
(Solar Superstorms Video)
A fury is building on the surface of the sun…jets of super-hot gas…rising waves of fire.
Renay San Miguel: You may remember from ScienceMatters Season 1, our conversation with John Wise, the Dunn Family Associate Professor in the School of Physics. His work on computer simulations and visualizations of the birth of supermassive suns and black holes enables Wise and fellow scientists a chance to turn back the clock, to the beginnings of the universe.
(Solar Superstorms Video)
Journey into our star…to explore its inner workings.
Renay San Miguel: For this ScienceMatters episode, we go from the massive…to the microscopic.
JC Gumbart also creates simulations, but this School of Physics associate professor goes in the other direction; he works on the atomic scale, picturing the possible movements of atoms, molecules.
(Riken Supercomputational Life Science Molecular Dynamics video)
New computer programs developed to simulate complex behaviors on the K computer reproduce the movements of tens of millions of water molecules.
Renay San Miguel: This video from Japanese science research institute Riken shows a simulation visualizing those movements at the atomic level. It’s called molecular dynamics. Gumbart says the method started to be used in research in the late 1970s. As computational power increased over the years, the quality of simulations improved.
So what do molecular dynamics do for Gumbart’s research?
JC Gumbart: we’re trying to represent in as realistic a way as possible all the atoms involved in the system, whether that’s a protein or maybe a protein in a membrane, some collection of biological molecules.
Molecular dynamics is essentially using the principles of physics, particularly classical mechanics, Newton’s second law, F=MA, but just applying it to thousands of atoms simultaneously.
Renay San Miguel: Newton’s second law: F=MA; force equals mass times acceleration. The force being applied to an object is equal to its size and how fast its speed changes.
Thanks to powerful software, molecular dynamics simulations mix those forces and objects on the atomic scale, allowing researchers to study how their movements evolve over a set amount of time. Where can this type of research lead? Gumbart says it’s already showing some promising signs in battling antibiotic resistance. That’s a growing medical problem that’s estimated to add $20 billion in additional annual healthcare costs. In another case, his research team’s molecular dynamics simulations gave Colorado doctors a new direction to consider regarding a certain protein deficiency.
At the very least, these simulations give scientists like Gumbart a chance to approach problems in a new way. And given advances in computational power, more progress could be coming soon.
JC Gumbart: So what this is allowing us to do is simulate both larger and longer. So larger gives us access to more and more realistic systems. So like I said we’re on the million atom scale. Some people are pushing the envelope up to a billion atoms. And I think within my lifetime we’ll see it get to the point where you could simulate even an entire small cell, like an E. coli bacterium, which might be on the order of 10 to 100 billion atoms. And then on the time scale we’re still pretty limited in the tens of microseconds perhaps, but that’s also growing, and so that will allow us to simulate more and more biological processes on realistic time scales.
(Upbeat music)
(Klaus Schulten TED Talk video)
The living cell is made of many molecules, macro-molecules, in fact the living cell has as many macro-molecules as the United States has citizens, and that’s a very good comparison, because these molecules in a cell form a society. They assemble and they work together.
Renay San Miguel: That is the late biophysicist Klaus Schulten, considered a founding father of molecular dynamics, giving a TED talk in 2010. While electron microscopes get science closer to molecules, they can’t make them move or determine their destinations. Computer simulations, said Shulten, turn molecules into citizens of their own molecular society.
(Klaus Schulten video)
It is a computer that is actually today finally permitting us to see the citizens at work, the citizens that in some cases just build pipes, and in some other cases some amazing machines that take the genetic information and turn it into new proteins of the cell, or to harvest the sunlight to solve the energy problem of nature.
Renay San Miguel: Gumbart actually learned from Schulten while working on his doctorate at the University of Illinois at Urbana-Champaign.
JC Gumbart: So I had gone there, started in the physics program, initially thinking maybe I was going to do something that’s more traditionally thought of as physics, like condensed matter.
But I found myself continually gravitating towards the computational aspects of the project I was working on, the modeling of the experimental setup, for example. And that was already a sign that perhaps computation was for me. And then I took a biophysics class just as part of—as an elective, and I was just really hooked by it immediately. And so I started looking into what labs I could join. This was still in my first year. And I ended up joining the lab of the late Klaus Schulten there who was one of the leaders in the field of molecular dynamic simulations. And so then it really just grew from there, my interest and work in the field.
Renay San Miguel: I interviewed John Wise during season 1 of ScienceMatters about his deep space simulations, visualizations, and he says it’s like rewinding space and time to help research the origins of black holes and stars and where certain kinds of stars come from.
So I’m wondering what do simulations reveal for your research?
JC Gumbart: So that’s actually a very good comparison, in the sense that I think both of us view our simulations as a way to watch what’s happening on a scale that’s normally inaccessible to us. You can’t go all the way back in time to the beginning of the universe, and similarly with our simulations, they act as like a computational microscope giving us access to a small scale that’s inaccessible to experiments, or at least under realistic natural conditions.
Beyond just, you know, sort of setting it—letting it go and seeing as it evolves in time, we can make perturbations, we can apply forces, we can, say, model a mutation.
Just to give an example of this: We recently published a paper—or we were involved in a team that published a paper in the Journal of Experimental Medicine a month ago where they had found, in a patient at the University of Colorado-Denver Medical School, a severe amino deficiency caused by a single-point mutation in a protein. And we modeled that mutation and were able to suggest why it may lead—it may compromise the protein’s function, leading to this deficiency.
Renay San Miguel: You’re not just looking for solutions to particular issues or applications for what you’re learning. You’re just trying to fill knowledge gaps also in how proteins work in all that?
JC Gumbart: Yes. And you know, the human genome codes for on the order of 20,000 proteins, and you know, we’d like to understand how every single one works.
Renay San Miguel: And that was something that only came to fruition what, last decade? Something along those lines, thanks to Craig Venter and those folks?
JC Gumbart: Yes, that was about two decades ago when they first sequenced the genome. But like many times in science, what was initially seen as perhaps the pinnacle of achievement was really just the starting point, and now we’re trying to untangle everything we can learn from the genome, how it leads to the proteins and how those lead to function.
(Upbeat Music)
Renay San Miguel: All kinds of organisms evolve, from humans on down. Unfortunately, for the last two decades, that includes disease-causing bacteria that have grown to resist the most commonly used antibiotics.
Molecular dynamics simulations have helped Gumbart and other scientists to understand what goes on inside these bacteria, especially what are called gram-negative bacteria. Gram-positive bacteria have one cell membrane; gram-negative have two, making it extra tough for antibiotics to fight through.
Gram negative bacteria are behind some of the most common infections, such as e. coli-related illnesses, pneumonia and gonorrhea. That’s because they usually develop something called efflux pumps, insidious defense mechanisms designed to keep you sick longer than you want.
JC Gumbart: I like all our projects, but one of the most exciting ones is looking at the multi-drug efflux pumps of bacteria. So in particular gram-negative bacteria like E. coli or Pseudomonas. They—one of the innate resistance mechanisms of these bacteria is that they express these efflux pumps which pump antibiotics and other harmful molecules out almost as fast as we can give it to them.
Renay San Miguel: The molecule itself is doing this?
JC Gumbart: Well, the efflux pump is a protein—is a complex of proteins, actually, that sits between the inner and outer membranes of these gram-negative bacteria. And so as antibiotics come in, they get pumped right back out by this preventing of buildup and keeping the bacteria alive.
And so we’re actually working with a team including Helen Zgurskaya at University of Oklahoma, also Jeremy Smith and Jerry Parks at Oakridge National Lab, to model both the assembly and function of these pumps and then also look for new small molecules that could inhibit their formation. And so in that sense, it would be like a one-two punch if you could knock out the efflux pump what our collaborator Helen has shown, is that then the bacteria become resensitized to existing antibiotics which they might otherwise be resistant to.
We’re looking at it from lots of different angles. So like I mentioned the one about efflux pumps, one of the innate resistance mechanisms. We’ve done a little bit of work on, for example, macrolide antibiotics, which bind to the bacterial ribosome and thinking about ways they can be possibly modified to evade resistance there.
And then finally, also, looking for new targets. So one of the main projects in the lab is looking at how outer membrane proteins get made by these gram-negative bacteria. It’s a protein system called the “BAM complex,” and we’ve been involved in that effort since the—really since the first structures came out, now about 6 years ago. I was involved in that work.
And so there, these are proteins that are absolutely necessary for bacteria to grow and to survive. And so, if we understand how they function, then we can possibly make suggestions for small molecules that can inhibit them. That could be a new—source of a new class of antibiotics.
(Upbeat music)
Renay San Miguel: Proteins that may be behind the development of diseases like Alzheimer’s and Parkinson’s are also on Gumbart’s radar. These diseases show abnormal protein buildups, or plaques, that drape nerve connections and cells with sticky deposits.
JC Gumbart: Atano Acharya is a post-doc I just hired in the last few months. He actually came on board with a project that he’s been working on for a while looking at the formation of these helices in Alzheimer’s plaques, so these fibrils. And so they’ve actually—they have developed a small molecule that—he and his previous lab—that they think may inhibit the formation, and so that’s something we’re starting to look at now.
So to be clear, it’s actually—Alzheimer’s disease is really quite a conundrum, in that so far every attempt to prevent it using our currently accepted model for how it comes about has failed. And you see, even just in the last couple months, drug trials getting to, say, phase II or phase III even and failing. So there’s still a lot of uncertainty surrounding it, but I think we have to keep trying things.
JC Gumbart: Perhaps one thing to just highlight is that our models are getting better and better. So there’s always some uncertainty when you’re trying to model a system from nature. You know, we’re going to get some things wrong, some approximations. But really, the field has reached a level of maturity that even wasn’t present when I started this about 10 to 15 years ago. Because of this, because of this maturation, in terms of the quality of our models, it’s becoming increasingly accepted by experimentalists. Our results are increasingly in agreement with theirs. And so we’re really finding this nice synergy developing where we can both explain the results from their experiments where they may not have the detailed information that we can access, and we can also predict the outcomes of new experiments based on our simulations.(Upbeat music)
In addition to his work in the School of Physics, JC Gumbart is a member of the Petit Institute for Bioengineering and Bioscience. His research is currently funded by the National Institutes of Health and the National Science Foundation.
I’d like to thank Gumbart for his time. Check out his SimBac – Simulations of Bacterial Systems lab website at simbac-dot-gatech-dot-edu.
Our thanks to Riken Research Institute and the University of Illinois at Urbana-Champaign for the use of audio.
Siyan Zhou, a former research associate with the School of Psychology, composed our theme music.
If you like ScienceMatters, please subscribe to our podcast. You can find us at Apple Podcasts and Soundcloud.
This is ScienceMatters, the podcast of the Georgia Tech College of Sciences. I’m Renay San Miguel. Thank you for listening.
(Upbeat music)