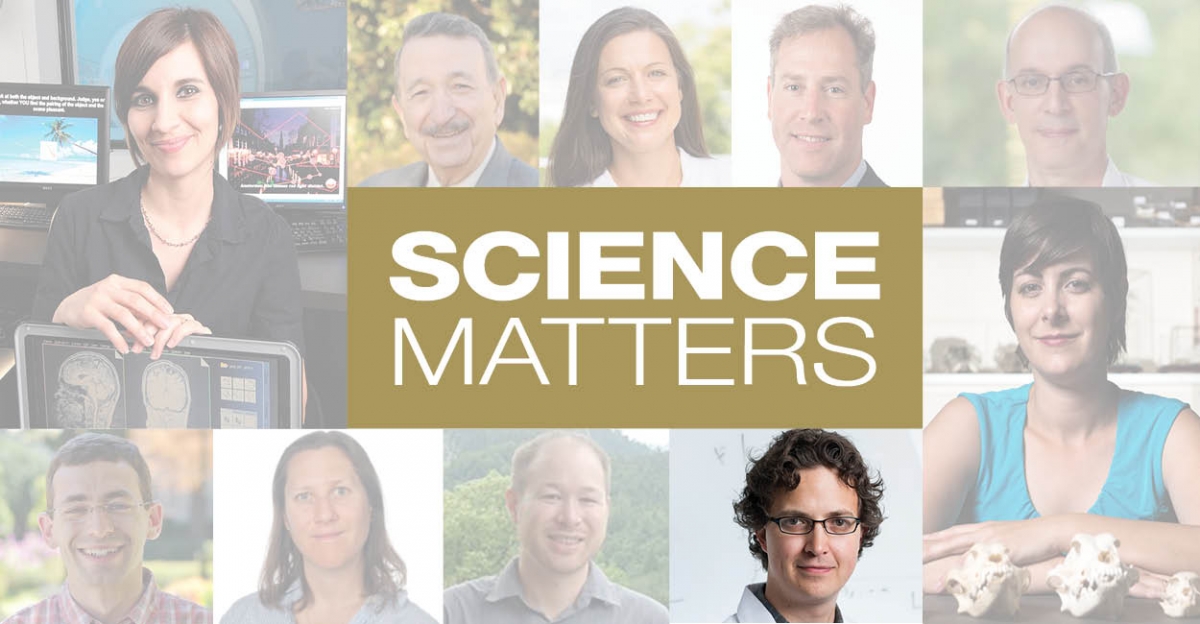
Download this episode
Simon Sponberg, a professor with joint appointment in the School of Physics and the School of Biological Sciences, studies how animals move through their environments. Among his unusual research methods is placing moths on joysticks so they can “play” video games in virtual reality.[Upbeat music]
- Renay San Miguel:
-
Hello and welcome to Science Matters, the weekly podcast from the Georgia Tech College of Sciences. I’m Renay San Miguel. Simon Sponberg loves to study how insects like moths and cockroaches move. The Georgia Tech professor discovers the physics and mathematics hidden within the biological systems of these creatures. And what he learns about animal locomotion could mean better robots, better prosthetic devices..better vehicles.
So yes, he loves studying how bugs move. What he doesn’t love..are the actual bugs themselves.
Simon Sponberg:
-
I would love to be able to tell you that I was one of those kids who like when I was six I went out and like there was a cool animal moving around and I was like, oh my god, that’s what I’m going to do for my life, but that was absolutely not the case. I had a traumatic experience with a cicada when I was six. I hate insects. In that sense it was really disturbing, but I’ve come to appreciate aspects of them…I’m not a natural entomologist, I wish I was. [Cicada sounds]
- Renay San Miguel:
-
So…just what was that traumatic cicada experience? You’ll have to wait a little while for the answer. Sponberg may not be a natural entomologist – that is, someone who studies insects professionally -- but he is an assistant professor in the Schools of Physics and Biological Sciences. He’s spent most of his career at the intersection of those disciplines as he discovers how creatures move on the ground or through the air, and what adaptations they’ve made along the way. Sponberg studies what’s called the physics of living systems. Here’s how Sponberg explains it in a Georgia Tech video from late 2017:
- Simon Sponberg video:
-
The control systems of animals are exquisitely tuned to the environment and the behavior that the animal is trying into execute in that environment, and I think one of the big challenges now is to figure out how the neural systems of animals, the mechanical systems of animals are working together in order to enable this really sort of exquisite level of adaptation and behavior that we see in the natural world.
- Renay San Miguel:
-
Sponberg wrote about the challenges and opportunities in that research for a September 2017 Physics Today cover story called “The Emergent Physics of Animal Locomotion.” In it, he writes of measuring the neural commands cockroaches send to their own muscles..of using robotic flowers to determine how huge hawk moths track their dinner…or having those moths play video games.
That’s right, video games. In virtual reality, even.
But we begin with a lizard -- the gecko -- and its superpower, one of the first aspects of the physics of living systems that Sponberg studied as an undergraduate at Lewis and Clark College in Oregon.
Simon Sponberg:
-
We were asking the question of how geckos stick to walls, and it’s very interesting because geckos will stick to almost any surface, and if you know much about chemistry, that's sort of hard. Things tend to be pretty chemistry-dependent, and so dependent on the particular structures of what makes up the gecko’s foot and the wall. There are a few things they won’t stick to like Teflon, and that ends up being a key to what's happening. So we were interested in how geckos are sticking to walls, and that sort of evoked my imagination of understanding not just how geckos could adhere, but how they could detach as well -- how they could actually do movement because if you’re an animal, sticking is really good until you need to be unstuck.
- Renay San Miguel:
-
You have to be able to move off that wall to get away from prey or whatever --
- Simon Sponberg:
-
If I only just stuck in one place for the entire time, that’s not so good, right? And they can actually attach and detach their feet in as little as forty-thousands of a second.
- Renay San Miguel:
-
Wow.
- Simon Sponberg:
-
So they can run quite agile-y despite using that adhesion, and they can stick by a single toe.
[Laughter]
And in fact, in a theoretical maxim, two 70-gram geckos could support my weight.
- Renay San Miguel:
-
But again, that’s theoretical; the gecko’s skeleton couldn’t support a person’s weight. But its tiny feet support its own weight while
hanging on a wall largely due to something called van der Waals forces, which is how two separate surfaces interact with each other on a molecular level, depending on their distance.
- Simon Sponberg:
-
If I stick my hand, put my hand on a table, I actually have lots of van der Waals forces between my hand and the table. But at the molecular level my hand and the table are very, very rough, and so I’m barely actually touching the table. And the gecko sort of overcomes this problem because he’s got little hairs on the bottom of his toes, and they split to a bunch of little split ends, and then those split ends split another ten, hundred, thousand times --
- Renay San Miguel:
-
And the can get traction, they can grab --
- Simon Sponberg:
-
They get so fine that the finest tip is smaller in diameter than the wavelength of visible light.
- Renay San Miguel:
-
Whoa.
- Simon Sponberg:
-
300 nanometers, and it basically allows them to make intimate contact with anything they touch.
- Renay San Miguel:
-
Oh man.
- Simon Sponberg:
-
And so they touch a surface, and if they orient their toes right -- basically they have to press in and slide a little -- then their hairs get really pressed up against the surface, and then adhere with van der Waals. But then if they change the orientation of their foot, they start to separate, and as soon as they start to separate becuase of van der Waals forces, they detach really fast.
[Upbeat music]
- Renay San Miguel:
-
Sponberg’s recent research uses robotic flowers to study how hawkmoths zero in on food sources. 21st century robotics, and some old school mathematics, help him decipher all the complex biophysical behaviors in these flying insects.
Simon Sponberg:
-
These are really large moths about the size of my hand, and they can hover in mid-air, and hovering in mid-air looks like one of these peaceful, calm behaviors. It’s actually dynamically unstable. What that means is that if the moth doesn’t actively detect something about its movement and do something every couple of wing strokes, it’s going to crash. So I now know in the context of that moth hovering in mid-air, I don’t have to ask a question of, ‘what does its brain do?’ I can ask a question instead of, ‘what does its brain have to do?’ and that sounds like a very similar question but it’s a little different and I'll explain. What I mean is if I know about the physics of the moving moth, and how if it gets buffetted by a little bit of wind how it gets knocked off balance --
- Renay San Miguel:
-
Sure
- Simon Sponberg:
-
-- now I know if it stays hovering, I know from the physics of how it moves that it has to be its brain that’s updating the mechanics and producing the force to stabilize it. So we can say, instead we can ask, ‘how does the brain stabilize the moth?’ or ‘how does the brain let the moth actively track one of these flowers in its environment when it’s moving around.
- Renay San Miguel:
-
When it’s doing that, is that a function of adaptation, adapting to its environment? Haven’t you also studied the hawkmoth’s eyes and how it, and the role they play in all this?
- Simon Sponberg:
-
Right. So in order to respond to the environment, the moth has to take in information about its environment, and then use that information in order to stabilize itself. And one thing that we’ve been doing is we’ve been exploring how that’s done. And so basically what we do, we make a bunch of games for our moth to play, and one of the games we make is, we make a little robotic flower, and we put a little nectar on the robotic flower that the moth can feed from. And you might say, ‘Simon, why aren’t you just using real flowers?’ And the moths will feed from real flowers, but when we have a robotic flower now I can very precisely and repeatedly control what the flower is doing, and that allows me to, in a dynamic sense presciribe what the moth is responding to, and so I can very repeatedly measure how the moth responds to the same input.
So we have these robotic flowers that wriggle around. It turns out the moth can track these flowers up to ten times a second wiggling back and forth, which means the moth can go back and forth, back and forth, back and forth, back and forth, and change its direction up to ten times a second in mid-air while it’s hovering, which to me is sort of remarkable behavior. But again it’s easy to say biological systems are complex, so how do we sort of understand that?
It turns out not just are they doing that sort of an impressive behavior from how they move, they actually have to see that flower, and they typically do all this behavior in light levels where you would have a hard time seeing the hand in front of your face. So at light levels that are below dusk levels, typically bright starlight kind of thing -- that’s actually where they prefer to forage because they’re nocturnal, or crepuscular animals is the technical term, they’re twilight fliers -- and so they’re out there foraging around in their flowers and they go up to feed from a flower, and they don’t land on the flower, they stick this long proboscis that they have curled up like a party favor in their mouth, and they stretch it out and they stick it into a flower and they feed from it, and the feed from it while they’re hovering in mid-air, so in fact there are many people, when they see these animals think their hummingbirds because they’re about the same size. In fact they use very similar physics.
So they’re hovering around and feeding from these flowers. So one of the questions we had is, how do they adjust to this low light level? Because they also fly in brighter light conditions. And this is an interesting challenge from a sort of a biophysics perspective, because light is, may be, the most variable quantity you deal with on a day to day basis. You don’t notice it because your eyes are pretty good at adjusting for light, but the hawkmoth’s eyes are incredible at it.
- Renay San Miguel:
-
The hawkmoth’s eyes, brain, and wings are all reacting to feedback – with the animal using that feedback to determine what works for it, and how it will move in the future. Sponberg believes researchers have to delve deeper into this feedback to learn more about animal locomotion. He is now able to design feedback into his experiments, sometimes in the most unexpected ways.
- Simon Sponberg:
-
Here’s the weird thing about the flower. If you think about it, the moth is not seeing the flower’s motion. The moth is seeing the flower’s motion relative to its motion. It's own motion. So if the flower moves one centimeter to the right, and the moth moves one centimeter to the right, now the moth says okay I'm just right in front of the flower, so what the moth cares about is the deviation between the two, and that’s actually what its visual system sees, and that is a function of the feedback loop of its motion to the flower’s motion. And this is one of those, and in fact if we had not done that appropriate feedback in our models we would have entirely made the wrong predictions. It was only by doing that that we were able to make these very precise predictions, so feedback is something we don’t study all the time in dynamical systems and feedback in physics, but this is very important here. And there’s other longer term feedbacks like what you're talking about which is learning, and that’s something that we’re setting out to start studying right now in the moth, and what happens -- how does it adjust over time if it’s living, if it starts struggling, and one of the ways we’re doing that is we’re making moths play video games in virtual reality. [Video game music]
- Renay San Miguel:
-
No, we don’t think Sponberg’s moths will be enjoying Super Mario Brothers anytime soon. But by tethering the moth to a joystick of sorts, Sponberg scores bonus points by using virtual reality to adjust what the moth is seeing in the experiment, and then measuring its movements and recording how it adapts.
- Simon Sponberg:
-
In virtual reality you can change the physics the animal experiences, okay? So what you can do -- think about it for a second. If you imagine you’re sitting there, sit there for a second. Now turn 90 degrees to your right.
- Renay San Miguel:
-
Um-hmm.
- Simon Sponberg:
-
That's – visually, that’s like if I picked up the entire world and moved it 90 degrees to your left, and you sat still. So In virtual reality, what we can do is we can say okay, well what happens if you’re sluggish? What happens if you suddenly – which is suddenly like what happens if increase your mass all of a sudden, which happens to a moth. They eat about fifty percent of their mass in about a minute. So we can say okay, well now if the moth tries to turn 90 degrees to its right, we’re only going to move the world 45 degrees to its left, and we can ask questions about how it learns to compensate for these changes and how responsive the world is or what the physics of the world is around it.
- Renay San Miguel:
-
It's got a heavier body, it has to navigate through all that, and you’re going one direction, and it’s starting to go another --
- Simon Sponberg:
-
A virtually heavy body, right, and so what that -- to go back to what we were talking about, right? We were saying what I’m interested in, and I think one of the major principles of organismal physics is asking these questions of how do you get behavior out of the interaction of the brain and the muscular system and the physics of the body, and the physics of the environment around it, like the air around the moth, and one of the really good ways to do that is to change one of those and look how the others compensate.
- Renay San Miguel:
-
Sponberg believes using robots, or robo-physics, along with the study of feedback will get researchers closer to answering questions about how animals move. And the potential applications of that research?
- Simon Sponberg:
-
If we understand that better, we typically are going to start being able to engineer better systems, so what happens is in robo-physics and biologically-inspired robots and such, we are trying to understand biological systems or physical mechanisms using robots, and along the way, lo and behold, we tend to make a lot of new insight into how to design robots, so that’s a good example. Another thing is if we’re leraning how the brain typically works with a body, that has very natural applications to neuroengineering, prosthetics, both of which are very big strengths here at Georgia Tech. So I'm actually partially -- I have a adjunct appointment in biomedical engineering for exactly that reason. I have a bioengineering student working in my lab whose trying to understand the mechanism of how these insect wings beat at high frequencies.
And it’s not because we think that like, what we really need is more robotic insects flying around, that’s not the major role. The major idea is that the mechanism they’re using is enormously useful from a power perspective and a speed perspective, so insects have solved this challenge of being both fast and using relatively low power, and that’s something that we would just like to understand how the heck does that work.
- Renay San Miguel:
-
And everything – all the research that you’ve conducted, all the work that you’ve done, has it helped you deal with that traumatic cicada incident from your youth?
- Simon Sponberg:
-
[Laughter] I appreciate those animals very much now. The experience that I had was -- I was actually, I saw these, I was living in New Jersey at the time and one of the major cicada events hit, so there were a bunch of shed exoskeletons that I was going out and picking them up and looking at them and such, so maybe I was pretty curious in them -- don’t know I don’t quite remember, I was six -- but one of them hadn’t fully separated from the cicada --
- Renay San Miguel:
-
Ohhhh..
- Simon Sponberg:
-
So I reached for it, and the eyes lit up, and I have this sort of nightmarish vision of, like, red eyes looking at me, and then the sound going on, the cicada sound is very loud. But boy do I appreciate that now, because how do you make that sound when you’re that small?
- Renay San Miguel:
-
Yeah, and it’s a loud sound too
- Simon Sponberg:
-
It's that loud, and it’s a very high frequency, so how do you do it? So these kinds of questions -- how do organisms do this kind of thing, especially – look, when all these amazing behaviors and motion and sound and perception that is available in the biological world, and I think the really exciting thing now is that there is a lot to be done from a physics perspective with respect to those things today, given not just with the tools, but with the perspectives that we have. [Upbeat music]
- Renay San Miguel:
-
That’s Simon Sponberg, assistant professor in the Schools of Physics and Biological Sciences. The website for his Agile Systems Lab studying the physics and physiology of animal motion, is at s1-dot-sponberg-dot-gatech-dot-edu. His Physics Today cover story, “The Emergent Physics of Animal Locomotion,” can be found in the September 2017 issue. I’m Renay San Miguel, and you’ve been listening to Science Matters from the Georgia Tech College of Sciences. [Upbeat music]