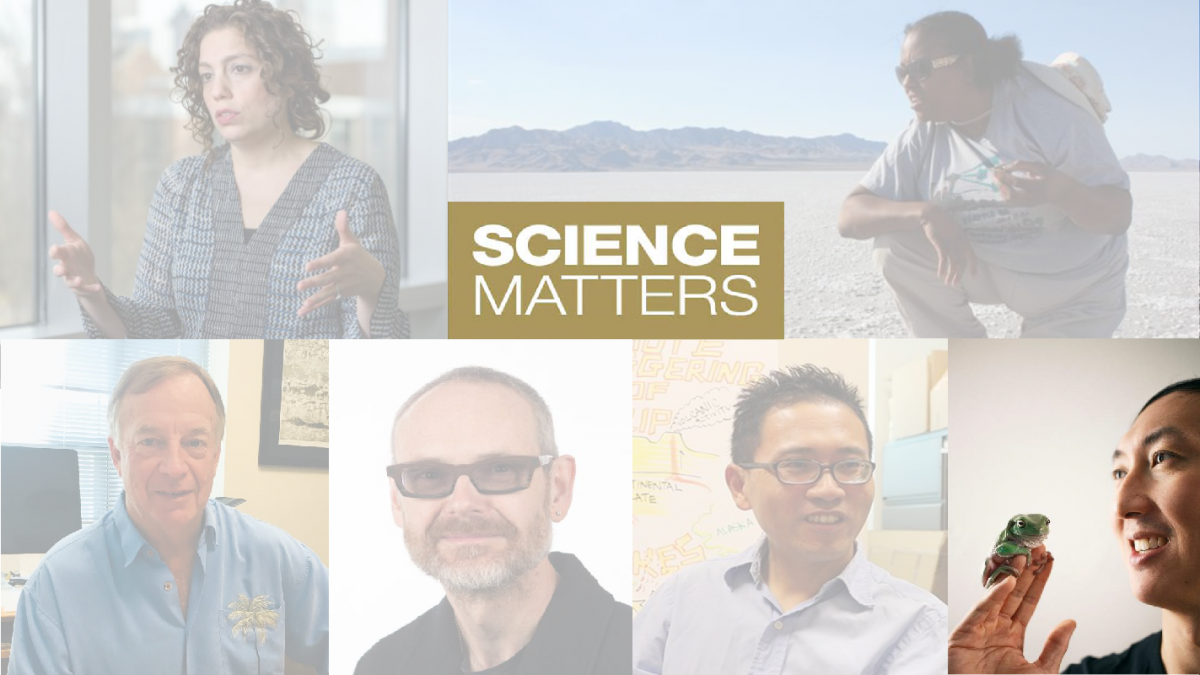
Download this episode
His incessant curiosity inspires David Hu to search for the physics among water-walking geckos, bridge-building ants..and urinating zoo animals. Hu, an assistant professor in the School of Biological Sciences, has a joint appointment with the George W. Woodruff School of Mechanical Engineering. This conversation is an edited excerpt from the Uncommon Engineer podcast. (Our thanks to Steven McLaughlin, Dean of the College of Engineering.)
(Upbeat music)
Renay San Miguel: Hello and welcome to ScienceMatters, the podcast of the Georgia Tech College of Sciences. I’m Renay San Miguel.
At Georgia Tech, we’re all about collaboration among our colleges and schools. Interdisciplinary cooperation happens all the time. That goes for podcasts as well.
The College of Engineering podcast, The Uncommon Engineer, recently published a lively episode with David Hu, associate professor in the George W. Woodruff School of Mechanical Engineering. He is also an associate professor in two College of Sciences units: the School of Biological Sciences, and the School of Physics.
That’s why we asked the College of Engineering if we could share excerpts from its conversation with Hu. It graciously agreed.
Hu’s studies of animal biomechanics routinely receive a lot of interest from the country’s top media outlets – especially when he focuses on unusual topics, such as frog’s tongues and saliva, how fire ants build water rafts with their bodies, and how long it takes certain animals to urinate. That latter study won Hu the 2015 Ig Nobel Prize, given annually by the group Improbable Research for scientific research that, as the group says, “first makes people laugh, and then think.”
The answers to Hu’s questions about animals – whether they are about bodily functions or the way they move -- have implications for engineering and medicine. Above all, his discussion with The Uncommon Engineer’s host Steve McLaughlin, Dean and Southern Company Chair of the College of Engineering, shows how Hu’s curiosity frequently gets the best of him, all to the benefit of science.
Here’s David Hu with Dean McLaughlin:
Steve McLaughlin: You know, when a lot of people think about mechanical engineers they think about trains and planes and automobiles and manufacturing and materials, but you study animals. Why is a mechanical engineer studying animals, and how does that apply to mechanical engineering?
David Hu: I'm a mechanical engineer, but I also, in mechanical engineering, I study a field called fluid mechanics, which is the motion of stuff like air and water. Lots of devices based on this, like ships and airplanes. But you know, the very first role models for us mechanical engineers and fluid mechanists were these things like birds and fish. Basically, nature's way to move around in fluids. They've been an inspiration for hundreds of years, but recently there has been technologies like high-speed cameras and 3D printing and 3D scanning that we can actually start to really figure out how these animals are doing these amazing things. And then when we look at these animals, we are hoping that we can use the principles that we see to design new kinds of devices.
Steve McLaughlin: You've gotten a lot of media coverage for some of your work—a splash, can I say—from a study that you did not so long ago about mammal urination. Can you say a little bit more about that, why you got started on that and what it is we can learn from it?
David Hu: Well it all started when I was changing diapers for my son and I had this— This thing happens when you change boys’ diapers. Sometimes they’ll actually just pee on you.
Steve McLaughlin: I’ve been there.
David Hu: And one of the things you noticed when you watch babies pee is that they take an awfully long time. At first, I thought that he had bladder problems, so I watched him. It took about something like 21 seconds. It takes a really long time. And if you go to the bathroom and time yourself, it's about the same. And that's just a very strange— This just aroused curiosity. I mean, I thought that maybe my son had some kind of health problem, because he weighs 10 times less than me, so I should have 10 times as much urine as he does. But he’s—
Steve McLaughlin: His bladder’s got to be one quarter or one 10th the size of your bladder.
David Hu: It turns out it's all proportional, so yeah, a kid’s bladder was a 10th, but it was coming out at the same time and it didn't make any sense. So I recruited some engineers to come try to understand the fluid mechanics of the bladder, and it turns out if you look in the history of this field of urology there's been a huge amount of questions of how the bladder works and how actually fluid comes out. There's controversy over why, if it is gravity driven or you have this special muscle called the bladder, is that pushing it out? And no one really knew. So this is a great example of turning to nature to sort of understand this thing.
So, since humans evolved from all sorts of different animals we actually went to the Atlanta Zoo and we measured the urination time of all these different animals. It's a huge range. Best one to give you an example of is an elephant. So to measure the urination of an elephant, students have to wake up at 5 a.m. when the Elephant just gets up, because elephants don't listen to anything you say. And then they go and put a huge kitchen garbage can underneath this elephant, and the elephant fills this garbage can. It's about 20 liters in about the same time as my son, about twenty-one seconds.
Basically, all these animals have the same thing in common. I figure there's got to be some common principle, because this is not normal. And so it turns out if you look into the anatomy, what the elephant has and what we all have is this special pipe. My kids and I, we call it the pee-pee pipe, but it's called the urethra. And for elephants, it’s a meter long. Little do people know that males and females both have these pee-pee pipes. For female elephants, it’s a meter long and is about the width of my fist.
What the elephant does and what the rest of these animals do to get urine out is that— the wider the pipe, you can imagine, it's like a highway. The more simultaneous lanes cars can go down. So the wider it is, more fluid can come out. But then the second effect is the fact that this pipe is really long, and that uses this Bernoulli effect, where basically if you have got a long pipe you can actually focus the force of the gravity so you can get fluid coming out a lot faster. You can notice this if for example you take—I think some students call it beer keg physics—if you take a beer keg, if you tap a hole in the middle, it will flow out way slower than if you tap a hole at the bottom.
And that's just an example of, you know, where you put this hole makes a huge difference in the speed, and that's basically what the animals have done. They've taken this like really, really thin pipe and infinitesimal amount of fluid and they use that to accentuate the speed so that even if you've got 20 liters of smelly urine, you can get out the same speed as if you only have like a few ounces.
So I started this thing as a curiosity, but it was— it just became— it just became a lot of fun. And it turned out that actually a lot of people, a lot of physicians and engineers, have actually been starting to use our discovery. There's this Japanese doctor who read about our work and he was— so this urologist, he interviewed 2,000 Japanese people all the way up, you know, from kids to adults. And he basically extended our rule—what we call the law of urination, this 21 second rule—and he said he found out that just because of natural aging, basically if you're 80 years old, you're going to urinate at an average time of 30 seconds instead of 20 seconds.
And before this, doctors, when they wanted to measure the health of the bladder, they had to use basic lasers or ultrasound. But now if they want to find out if your bladder muscle is weakening, they can just measure your urination time and show that, oh, your bladder muscles are really weak. I was really happy to see them see this and use our discoveries to sort of help older patients.
There's also the example of— they’re make making prostheses, basically these sort of artificial urethras. And they needed basically a way to test these devices to make sure that they're sort of robust enough to last for a lifetime. And so now they've made it the official protocol for testing urethras is this 21 second rule urination. They'll make sure that it can urinate for 21 seconds every few hours and they'll do this test for days and days and days on these on these products. I mean, I was really happy to see that doing something that was fun could actually influence, you know, scientists from the Netherlands all the way to London and all over the world.
Steve McLaughlin: Let's talk about the frog tongues. Some of your more recent research, again really, really cool stuff. You know, I think as kids we've seen frogs using their tongues to grab stuff. What can we learn from a way a frog uses its tongue for engineering?
David Hu: So this frog tongues project started when I was teaching a class on biology at the Atlanta Botanical Garden. I was just really interested in how they do it. So the first thing we did was we took a high speed camera of a frog. And it's amazingly fast. It can reach out and grab an insect in a thirtieth of a second. It could fit 10 frog strikes in a single eyeblink. And we looked around and we realized that no one really knew why the tongue could grab something so fast. And it seemed like it would have pretty obvious applications, like if you have these micro air vehicles, if they want to actually pick up a cargo, if they want to pick it up before it leaves, they've got to really move fast too.
So this is an example of reverse engineering how the frog tongue works. So I hired this graduate student named Alexis Noel. She became famous for carrying around a bag of bloody tongues. Because one of the very first things that we did was we got tongues from biomedical engineering. One of their dissection classes had these frogs and we got 20 frogs and we opened their mouths. The frog— this is actually the first time I ever touched a frog tongue. The thing I found out it is amazingly soft. It turns out it's 10 times softer than the human tongue. It's like 20 times softer than a marshmallow. In fact, the only material out there that's as soft as the frog tongue is basically the human brain. Nature has basically not come up with anything really softer. That's about as soft as it gets.
If you touch the frog tongue, it kind of feels like a sticky chewing gum, but it has this weird sort of sticky coating. And this coating, if you take it off with your fingers and slide it between your fingers, it feels really weird. It's sort of sticky at first, but then when you slide harder it gets slippery. So this is the property of materials called non-Newtonian fluids. A typical example of this kind of fluid is like paint. So paint was engineered by chemical engineers who wanted a material that— Imagine you put paint in a can. You can stir it with your brush. But then when you put it on the walls, it's got to stick.
So in other words, frog saliva, just like paint, it kind of has like an on-off switch. When you push it really hard, it flows like a liquid, but then when you push it softly it sticks. It just sort of holds its grip. And that's exactly how the frog saliva and tongue work. It's got to be really soft and have this special sort of on-off switch in the fluid. So when it reaches out and grabs the prey, it's able to stick, and then when it pulls it back to its mouth it actually uses these eyeballs that move ultrafast and they push the prey off the tongue. So it's got to be able to sort of stick to the prey and then make sure the prey can come off later.
Steve McLaughlin: We talked about tongues, we talked about urination, and I know one of the things that at least I saw was after the flood in Houston, your work on ants and how ants respond to their anthill being inundated with water, or what they do in a flood, and you got a lot of publicity around that. Can you tell us a little bit more about your work with ants and how it relates to floods?
David Hu: Have you ever gotten bitten by a fire ant?
Steve McLaughlin: Oh yeah. Yeah.
David Hu: They're a pretty big deal all over Georgia, and in fact they’re a species that’s invasive to the entire half of the United States. They're spreading all over the world. I was just, a week ago, I was just talking to a radio station in Korea. They were just freaked out seeing fire ants show up at their harbor, and they were worried that they were going to end up kind of like the United States.
And so why would an engineer want to study fire ants? Fire ants are interesting because they can actually survive floods. And that's not typical for an insect. Most insects— There’s these insects called water striders, these insects that sort of— They kind of like, on the surface of lakes, they glide. They're really elegant. They move quickly. Those are the one of the few exceptions. But most insects that stuck in water, they usually just become food for water striders and they drown. But these fire ants, they're one of the few species that actually can survive flash floods.
There are about 10,000 species of ants in the world, and this one evolved in Brazil in this area called the Pantanal. It's a sort of vast wetland that every single year during the rainy season it floods up to a meter deep. Any animal that had to survive this had to evolve ways to deal with water. And so what the fire ant does, it does this amazing thing. If you— and you can actually simulate this. If you have fire ants in your home, you can take a bucket of them, and you just drip water into the bucket. And if you do it slowly enough, you don't drown the ants. The ants will actually come out of their underground homes and what they'll do is link themselves together, and they're going to build this waterproof raft.
By linking their bodies together, they can actually trap air pockets, and that makes the entire thing really buoyant and it makes it really pretty much seaworthy. And as a result, these sort of dinner-plate-sized ant rafts, they can float out to the open ocean, they can last for months just by feeding on their sort of offspring. I know that's kind of gross. They're really, really good at being waterproof.
What we discovered in our lab was that they're not just good at being waterproof, but these rafts, they’re kind of like— they’re an example of— I mentioned this earlier, but is a non-Newtonian fluid. They're an example of a material that's both kind of liquid and solid at the same time. For example, these rafts sort of strike— you can you can take an ant raft and you can squeeze it between your hands and it’ll sort of spring back out, kind of like Silly Putty. Or you could sort of drop a rock on top of it, and instead of the raft sinking the rock will actually sink. The individual ants will sort of feel the presence of the rock and they'll move out of the way and reconnect on the other side so that the rock actually kind of penetrates the raft like some Terminator material.
It's amazing that the ants can do it because they're living things, and they do it purely by mechanical connections. So they just basically make and break these connections and they can sort of have these properties that make them really resilient. And so this project was actually funded by the Army Research Office. They were really interested in self-healing materials, and I mean ants can sort of give us some kind of idea of how to build a really self-healing material, just like ants can.
(Upbeat music)
Renay San Miguel: Again, that is David Hu, associate professor in the Schools of Physics, Biological Sciences, and the George W. Woodruff School of Mechanical Engineering. His first book, How to Walk on Water and Climb up Walls: Animal Movement and the Robots of the Future, was published in November 2018.
We thank the College of Engineering and its dean and Southern Company chair, Steve McLaughlin, for sharing their awesome Uncommon Engineer episode with ScienceMatters. McLaughlin is also the Uncommon Engineer’s host.
If you like listening to ScienceMatters, please subscribe to our podcast on Apple Podcasts or Soundcloud.
You’ve been listening to ScienceMatters, the podcast of the Georgia Tech College of Sciences. I’m Renay San Miguel. Thank you for listening.
(Upbeat music)